Introduction
The monograph 'Inflammation: clinic and therapy' (Part I and II), published by 'l'Oculista Italiano', brings together numerous relevant scientific contributions highlighting the importance of the inflammatory component in various ocular diseases.
The two volumes describe for each pathology the current pharmacological, preventive and/or curative treatment for the therapeutic resolution of inflammation involving both the anterior and posterior segments of the eye. The efficient resolution of the inflammatory response is indicated by the successful outcome of the treatment as reflected by the protective effect of the compromised tissue, the removal of debris and dead cells and the return of damaged tissue to homeostasis (Fig. 1).
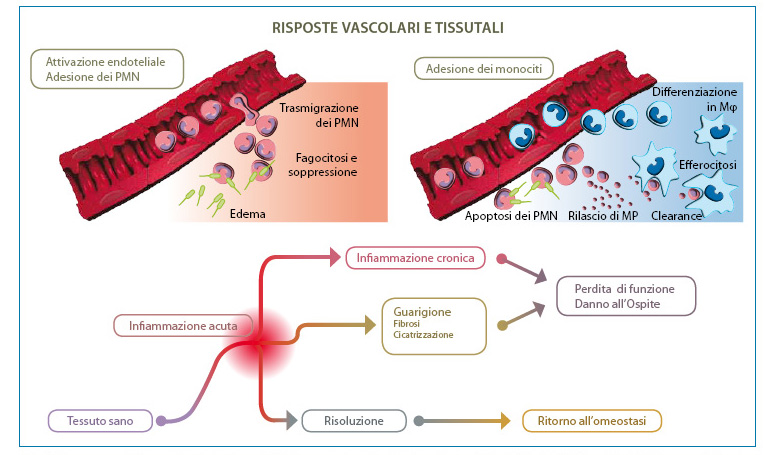
It has been further confirmed how the inflammatory process involves a complex cascade of molecular and cellular biological signals, that alter the physiological responses of the ocular tissue concerned.
The monograph also clearly shows how phlogosis is a transversal process which, when triggered by an injury event, results in particular clinical symptoms, not only confined to ocular pathologies frequently associated with inflammatory processes (e.g. allergy, conjunctivitis, keratitis, uveitis, etc.), but also, as has been demonstrated in recent years, to pathologies such as glaucoma, AMD and diabetic retinopathy.
New revisions emerge from the monograph by several authors also on the inflammatory aspects related to ocular surgery e on restoring tissue function. At the site of the lesion, cells release molecular signals that cause a number of changes in the affected area: dilation of blood vessels, increased blood flow and vascular permeability, exudation of fluids containing proteins such as immunoglobulins, and invasion by leucocytes. Several types of leucocytes, including granulocytes, monocytes and lymphocytes, are involved in the inflammatory process.
Continuing progress in the knowledge of the biochemical and cellular pathways associated with the inflammatory response has made it possible to enter the innermost mechanisms of ocular pathologies. The clinician thus has the possibility of using not only drugs that have already been clinically tested and consolidated (corticosteroids, non-steroidal anti-inflammatory drugs, anti-proliferative agents, anti-suppressants, biopharmaceuticals), but also of testing new pharmacological hypotheses for some more complex ocular pathologies thanks to which, also with the support of new drug administration methods, it is now possible to manage many ocular inflammatory processes of the anterior segment (e.g. dry eye, keratitis, conjunctivitis) and also of the ocular segment of the eye with sufficient efficacy. dry eye, keratitis, conjunctivitis) and also in the posterior segment of the eye (e.g. diabetic macular oedema, uveitis). However, although a broad therapeutic armamentarium is available, for some ocular pathological conditions, especially those characterised by high chronicity, the use of certain drugs poses problems of efficacy and/or toxicity. Chronic and prolonged inflammation is a defining feature of diseases such as atherosclerosis, obesity, diabetes, rheumatoid arthritis, asthma and various types of cancer. Many of these diseases have now established ocular manifestations in diabetic retinopathy, scleritis, uveitis, dry eye syndrome and ocular neoplasms. This also leads us to take a close look at the pharmacological and clinical advances that are being made in areas seemingly distant from ophthalmic application, but which could potentially, by translation, also be usable in the ocular field. Indeed, despite the fact that many ocular tissues have an immuno-privileged or highly evolved system to protect the delicate visual axis1-3 the essential control of acute inflammation is regulated by the same general pathways, mediators and effector cells that manage inflammatory responses in other organs. It is no coincidence that most of the immunosuppressants used in inflammatory eye diseases were originally developed in transplant medicine, for rheumatic diseases or other systemic diseases of inflammatory origin.
Recently, a significant alteration in the cytokine and chemokine profile was observed in aqueous humour samples from diabetic patients without any overt retinal pathology, but with a marked similarity to what was found in patients with overt retinopathy4. This scientific information not only lends further support to the fact that chronic inflammation plays a significant role in the pathogenesis of retinopathy, but also suggests that the inflammatory process is activated even before retinopathy (diabetic and/or proliferative) is clinically diagnosed. The alteration in the profile of certain cytokines could, therefore, become both a predictive marker, but also an important target for the development of future therapeutic options. In addition, the considerations described so far reinforce the hypothesis that although the current therapeutic armamentarium allows for the effective management of the inflammatory component of many ocular pathologies, the patient's demand for new diagnostic tests, new more effective and/or safer drugs still has ample experimental margins to be satisfied. In the remainder of this article, some hints on the pharmacology of inflammation will be taken up to point out some examples of the development of potential new therapeutic approaches that could, in the near future, prove to be alternative and/or complementary to those currently in use.
Pharmacological experimentation and clinical application
A hallmark of most inflammatory responses is the recruitment of polymorphonucleates (PMNs) to the activated vascular endothelium. An exception is autoimmune or allergic responses driven by lymphocytes, although lymphocytes or macrophages also initiate inflammatory responses (e.g., asthma, rheumatoid arthritis) characterised by a pronounced infiltration of PMNs or eosinophils into the damaged tissue. Indeed, excessive activation or dysregulation of PMNs can cause considerable damage to the tissue involved in inflammation, as seen in cases of ischaemia-reperfusion, corneal fibrosis and keratitis. More importantly, the early transient event in the inflammatory response due to PMN infiltration triggers the movement of macrophages towards the efficient removal of no longer active PMNs, a key step in the resolution of acute inflammation5 (Fig. 2). It is therefore evident how essential it is to understand the mechanisms of this process for the research and development of new anti-inflammatory therapeutic agents.6,7.
[caption id="attachment_1608" align="aligncenter" width="760"] Fig. 2. Helpful inflammation requires active resolution. Tissues experience frequent acute inflammation in response to injury, stress or infection. The recruitment of PMNs into the vascular bed is initiated and amplified by well-structured and organised pro-inflammatory circuits. The successful execution of acute inflammation requires phagocytosis of PMNs by macrophages, a non-inflammatory response. Phagocytosis of PMNs induces the formation of anti-inflammatory mediators to restore normal tissue function. Resident circuits in the cornea, uvea and retina, i.e. 15-LOX and/or HO produce autacoids that counter-regulate pro-inflammatory circuits, reduce PMN infiltration and activate macrophages for PMN removal. Dysregulation of this fundamental process sets the stage for the development of inflammatory disease. Modified from: Gronert K. 'Resolution, the grail for healthy ocular inflammation'. Exp. Eye Res. 2010; 91(4):478-85.[/caption].
Acute inflammation is an evolving dynamic process and as such also represents an 'unstable' state that may resolve or persist. In general, acute inflammation progresses towards its natural ideal outcome, which is resolution, with the neutralisation of harmful agents, cessation of PMN infiltration and functional tissue restoration (Fig. 2). Traditionally, ocular anti-inflammatory therapies have focused on strategies that decrease or neutralise the level of pro-inflammatory mediators and/or inhibit leukocyte activation. These therapies include non-steroidal anti-inflammatory drugs (NSAIDs), glucocorticoid (GC) receptor agonists and antibody or target-specific pro-inflammatory cytokine inhibitors, such as tumour necrosis factor alpha (TNF)-? and interleukin (IL)-1. In recent years it has been recognised that the therapeutic strategies based on pro-resolution are potentially effective in the treatment of multiple inflammatory conditions8-11. In reality, resolution is an active process involving innumerable mediators and different biochemical control pathways, and is carried out through:
1) Resolution of the inflammatory response;
2) switching from the generation of pro-inflammatory mediators to the production of pro-resolution mediators; 3) shutting down signal transduction associated with cytokine production and leukocyte survival;
4) Apoptosis of activated inflammatory cells;
5) phagocytosis of apoptotic cells (mainly by macrophages in a non-logistic process)
6) switching from pro-inflammatory to pro-resolution cell phenotypes (particularly relevant for macrophages).
Pro-lipid mediators
The discovery of a number of lipid mediators, derived from polyunsaturated fatty acids, as anti-inflammatory and pro-inflammation-resolving agents is mainly due to Serhan and his collaborators12-14. These include lipoxins, E-series (RvE) and D-series (RvD) resolvins, protectins/neuroprotectins and maresins. Resolvins, lipoxins, protectins and maresins are synthesised from arachidonic acid (AA) and the fatty acids eicosapentaenoic acid (EPA) and docosahexaenoic acid (DHA) (Fig. 3).
[caption id="attachment_1611" align="aligncenter" width="761"] Fig 3. Biosynthesis of lipid-prolonging mediators.[/caption].
Biosynthesis occurs as trans-cellular events, the details of which were recently examined by Serhan15. The use of lipidomics also allowed Serhan's group to identify and characterise new families of lipid mediators (Fig. 4).
[caption id="attachment_1610" align="aligncenter" width="750"] Fig. 4: Structures of the main pro-lipid mediators[/caption].
Pro-resolving mediators are bioactive lipids secreted in response to an inflammatory state and which stimulate molecular and cellular events to resolution. Lipid mediators are in fact able to promote a number of actions, such as blocking leukotrienes and prostaglandins, reducing cytokine release and monocyte recruitment, as well as reducing the nonphlogistic removal of apoptotic PMNs, which is essential for tissue homeostasis. Lipid mediators have also been shown to play a modulating role in several experimental animal models of acute and chronic inflammation of arthritis, peritonitis, ischaemia-reperfusion, pain and asthma16-22. Therapies that actively promote resolution may also have the advantage of improving innate immune responses caused by bacterial infections21. The possibility of treating inflammation without compromising the host's immune defences appears to be the most important feature that can be achieved through the administration of lipoxins, resolvins and protectins, or even better their stable synthetic analogues, as therapeutic agents in the management of various ocular inflammatory diseases. Taken together, therefore, the studies that will be mentioned are interesting for the development prospects for new anti-inflammatory drugs.
In particular, this new approach changes the traditional use of anti-inflammatory drugs from theantagonism (inhibitory therapy) to agonism (pro-inhibitory therapy). The advantage of using immune-resolving drugs is that they limit the continuous infiltration of neutrophils, counteract pro-inflammatory mediators, improve the containment and phagocytosis of cell debris and apoptotic neutrophils, and promote the restoration of tissue homeostasis.
Lipoxin
Lipoxin A4 (LXA4), lipoxin B4 (LXB4) and aspirin-activated lipoxin (ATL) were among the first mediators biosynthesised by arachidonic acid to be recognised as anti-inflammatory and pro-restorative lipid mediators23. In particular LXA4, the most studied lipoxin, binds with high affinity to the FPR2/ALX receptor24,25expressed in different cell types, including neutrophils and monocytes, and a regulator of some important functions of the immune response. Activation of FPR2/ALX by LXA4 leads to a reduction in leukotrienes, leukocyte migration, tumour necrosis factor (TNF)-induced chemokine production, chemokine receptor and adhesion molecule expression, pathogen-activated interleukin IL-12 production and superoxide formation, thereby enhancing nonphlogistic phagocytosis of apoptotic cells by macrophages26-28. These results also explain why the FPR2/ALX receptor is currently the focus of intense pharmacological screening for new anti-inflammatory agents.
It has also been shown that the autacoid lipid circuit activated by 15-LOX plays a key role in the generation of lipoxin A4 and is also present in the cornea, retina and uvea29-35.
In a recent study, it was established in particular that the 15-LOX and heme oxygenase (HO) systems work in concert to control the inflammatory response in the cornea31. Clear evidence of this activity was obtained using a knockout mouse model that, lacking these enzymes, produces amplified inflammatory effects. In this study, restoration of corneal homeostasis is achieved by topical ocular administration of LXA4.
It has also been reported that topical administration of LXA4 within the corneal tissue promotes re-epithelisation and is therefore able to reduce tissue damage after injury29.
The anti-inflammatory effect of topical administration of LXA4 on various pro-inflammatory markers was also demonstrated in an endotoxin-induced uveitis (EIU) model in rats36. In this trial it is shown for the first time that the topical application of LXA4 significantly stimulates the reduction of the inflammatory process in the anterior chamber of the eye. Furthermore, in the same study it is reported that topical treatment in rats with LXA4 (200 ?g/eye), when compared to animals treated with vehicle alone, also reduces the passage of inflammatory cells and protein extravasation into the aqueous humour induced by lipopolysaccharide. Lipoxin LXA4 is also more potent than prednisolone in reducing inflammation in the EIU model, and effective at a 20-fold lower dose. It is believed that the possible mechanism of this effect is due to the ability of LXA4 blocking the activation of the nuclear factor NF-kappa B and consequently inhibiting the production of pro-inflammatory mediators37. Recent experimental work also indicates that LXA4 exerts an important anti-fibrotic action, effectively helping to fine-tune the resolution towards tissue homeostasis38,39. It must be said, however, that the therapeutic use of LXA4 is severely limited by rapid in vivo metabolism and chemical instability. However, the process of synthesising stable derivatives of LXA4 is already active40 and molecules could soon be obtained from pre-clinical to be tested in clinical research, for new interesting therapeutic alternatives in the regulation of inflammation in various eye diseases.
Resolve
Table 1 shows41 some significant milestones on the study of the resolution of inflammation and the related links of experimental development of lipid mediators. Resolvins were the first pro-inflammatory molecules to be tested in the clinic.
Many of the anti-inflammatory activities of resolvins have been demonstrated in animal models of inflammation in the experimental treatment of colitis, periodontitis, acute kidney damage and peritonitis. In particular, resolvins show anti-inflammatory activity by reducing the infiltration of neutrophils and the expression of pro-inflammatory cytokines. In a model of allergic inflammation, RvE1, in nanogram quantities, promotes the resolution of inflammation in the respiratory tract. In part by suppressing the production of IL-23 and IL-6 and also by increasing interferon-? concentrations in the lungs of treated animals42.
In the ocular field, resolvins promote tear production, prevent the loss of integrity of the corneal epithelial barrier and inhibit the transformation of keratocytes to myofibroblasts. In addition, these molecules modulate the cellular response of T lymphocytes and reduce apoptosis of conjunctival goblet cells43-45. In particular, in experimental studies conducted on the Dartt rat, the secretion of conjunctival goblet cells, important components in dry eye disease and ocular allergy, is completely reduced by the resolution of inflammation triggered by RvD1 and RvE1. These good preliminary results encouraged the initiation of ophthalmic development of resolvins. Synthetic risolvine analogues [RX-10001 (RvE1) and RX-10045] are currently being evaluated in dry eye therapy46. Experimental evidence on the role played by resolvin RvD1 in regulating the ocular allergic response was recently acquired47. The study shows that the interaction of RvD1 with the GPR32 receptor activates48 signals that block the histamine-stimulated allergic response in conjunctival goblet cells. These positive preliminary results open up interesting therapeutic perspectives for resolvins in the treatment of ocular allergic conjunctivitis and other histamine-dependent diseases.
As is now well known, various mediators of inflammation are generated during the progression of choroidal neovascularisation involving the retinal pigment epithelium, Bruch's membrane and other retinal structures. It has also been observed that retinal endothelial cells under conditions of persistent inflammation release E1 and D1 resolvins, which is followed by a reduction in the expression of pro-inflammatory molecules and the inhibition of PMN transmigration across choroidal retinal cell barriers49. This is further confirmation of the beneficial role that potential analogues of resolvins could have as new therapeutic agents in degenerative inflammatory diseases of the posterior segment of the eye.
Proteins and tides
Proteins also play a key role in the control of inflammatory processes, as shown by experiments in animal models of peritonitis, ischaemia/reperfusion, asthma and stroke50-53. The anti-inflammatory effects of (neuro)protectin (N)PD1 are such that it protects retinal pigment epithelial cells from apoptosis induced by oxidative stress54- 56. In addition to the anti-inflammatory properties, the regenerative properties of NPD1 were also highlighted.57. The same study also hypothesised a potential role for NPD1 in the treatment of neurotrophic cornea.
Maresins, originally isolated from murine macrophage exudates, show anti-inflammatory activity with potency very similar to RvE1 resolvin and D1 protectin58,20. The anti-inflammatory and pro-resolving effects of maresins have been confirmed in several in vivo experiments conducted with the molecule called maresin 1 (MAR1) produced by human macrophages, which inhibits the infiltration of neutrophils in induced peritonitis in mice and enhances the uptake of apoptotic neutrophils. The data acquired so far suggest a potential application for maresins in the modulation of various aspects of the inflammatory process and in the resolution of pain and tissue regeneration58. These latter characteristics put the maresins in a position to be tested in the ophthalmic field (alone or in combination with other drugs) in those inflammatory pathologies in which the pain component plays such a predominant role that an adequate and specific therapeutic approach is required.
Other options for the development of new drugs with anti-inflammatory activity
An example of rational pharmacological development of new classes of molecules potentially useful in the therapeutic management of inflammation has been described in the previous paragraphs. Time will tell whether these new therapeutic strategies will have the desired clinical success.
Fortunately, pre-clinical experimentation has embarked on several avenues in the search for new and effective therapeutic solutions to combat various inflammatory diseases, both acute and chronic.
Chemokine antagonists
The immune system is directly or indirectly involved in numerous ocular diseases of the anterior and posterior segments of the eye: virus- and bacterial-induced keratitis, infectious and non-infectious (autoimmune) uveitis, dry eye syndrome, oncogenic events such as uveal melanoma, corneal transplant rejection. Other diseases include age-related macular degeneration, glaucoma, chorio-retinal degeneration and autoimmune retinopathy.
Inflammation is central in the development of immune responses, in both normal and pathological conditions, and there is now evidence that the complex chemokine system is not well regulated in this type of immune-dependent disease.
Chemokines, small peptides of 8-12 kDa with chemotactic action, are produced by different cell types at the sites of inflammation, and are involved in the adhesion of leukocytes in the activated endothelium and the subsequent transmigration and extravasation towards inflamed tissues. Among the families of chemokine receptors CXCR3 is probably the one that plays a decisive role in the development of autoimmune diseases as it produces, in certain tissues, cycles of local amplification of inflammation with relative worsening of the clinical pathological picture59. Consequently, CXCR3 has become a very promising target for the drug discovery which led to the identification and development of several antagonist molecules with potential clinical application in the treatment of chronic inflammation60,61. One of these molecules, called T487, reached phase IIa clinical development in the treatment of psoriasis. Unfortunately, the lack of efficacy caused its further testing to be suspended. This negative result, attributed to the molecule's poor receptor affinity for its target, has not affected the possibility of continuing to test this interesting therapeutic strategy.62. ?
The experimental study on the role of chemokines and their receptors in the pathogenesis of eye diseases caused by acute and chronic inflammation is rather new.
Recently, the expression of certain chemokines including CXCR3 has been determined both in the tear film and on the ocular surface of patients with dry eye and especially with Sjögren's syndrome63. Recently64Moreover, it has been shown that the ocular surface of mice lacking chemokine receptors is less susceptible to damage caused by inflammation due to the inability of CD4 cells+T-lymphocytes (T Helper lymphocytes, whose main function is to organise the immune response) to target this tissue in response to the stress of desiccation. This prevents the activation of the amplification loop that leads to chronic inflammation and subsequent aggravation of ocular pathology. These preliminary observations point to the therapeutic use of chemokine antagonists also in the management of inflammation in dry eye pathology.
Recently, a highly selective antagonist for the chemokine receptors CCR5 and CXCR3, the product called TAK-779, was successfully used in corneal transplants65,66. In this study, the product was injected directly into the stroma of the cornea.
Sennlaub et al. (2013) hypothesised67, for the first time, that in knockout mice lacking the CXCR1 receptor, the chemokine CCL2, also known as monocyte chemotactic protein 1 (MCP-1), increases in eyes with geographic atrophy. Furthermore, it was observed that CCR2 monocytes+ are particularly recruited in the atrophic lesion. The accumulation of CCL2 and CCR2+ is much more pronounced in mice lacking the CXCR1 receptor, which develop subretinal inflammation and photoreceptor degeneration. Inhibition of CCL2/CCR2+ could, therefore, represent an additional therapeutic target for the control of chronic inflammation in sub-retinal diseases such as AMD in both its atrophic and neovascular forms.
Phosphodiesterase 4 inhibitors
Phosphodiesterase 4 (PDE4) inhibitors and other therapeutic agents capable of increasing levels of cyclic adenosine monophosphate (cAMP) play a very important role in the immune system68. In general, these are molecules that stimulate suppressive effects on the deleterious actions exerted by inflammatory cells69. In recent years, there has been a series of experimental trials showing that cAMP is also involved in the resolution of inflammation70-73. In these studies it was reported that cAMP stimulates the transition of pro-inflammatory macrophages into inflammation-resolving macrophages. Furthermore, in experimental models of neutrophil- and eosinophil-mediated inflammation, it has been observed that the increase in cAMP stimulated by the administration of Rolipram, a selective phosphodiesterase inhibitor, or other cAMP-mimetic drugs, conclusively results in the resolution of inflammation. Recently, this potential therapeutic strategy in counteracting inflammation by specific cAMP-stimulating agents was presented at the ARVO 2013 congress. In this study74Through pharmacokinetic and preclinical studies in vitro and in vivo, it is shown that an aqueous formulation containing a PDE4 inhibitor, administered topically in the rabbit eye, is able to achieve sufficiently adequate drug concentrations in the cornea, conjunctiva and lacrimal gland. This preliminary study suggests that phosphodiesterase-4 inhibitors can be further explored as potential drugs in the treatment of ocular surface inflammation.
Cell therapies in the treatment of ocular inflammation
A radically different pathway from the pharmacological approach, described above, in the treatment of ocular inflammatory diseases could in the future come from cell therapy.
In recent years, there has been a growing interest in the possible application of cell therapy in certain eye diseases that have a major impact on the risk of visual impairment. Naturally, in this specific therapeutic treatment, as the eye is considered an immune-privileged organ, it is assumed that there is a reduced risk of rejection by the immune system. In a recent review75 those cell therapies that might have a high probability of being used especially in the field of intraocular inflammation are highlighted.
Cell therapy can be divided into two broad classes: one, through the use of stem cellsis aimed at remodelling the structure and relative functionality of specific tissues and cells; the other, using immune cells in the role of potential immunomodulators of inflammation, is directed towards restoring immunological homeostasis by controlling those harmful effects caused by inflammatory pathologies and which have been extensively described by several authors in the two monographic volumes 'Inflammation: clinic and therapy', published by 'l'Oculista Italiano'.
One of the main fields of stem cell research is aimed at restoring vision in patients with retinal degenerative diseases. The implantation of stem cells in this type of pathology has reached a very advanced stage of development76. Another ophthalmic field in which the technology and clinical use of stem cells has advanced considerably is the application of stem cells from the limbus corneal in ocular surface diseases. The eye presents a barrier to microbial invasion to ocular surface integrity in which the limbus represents the connecting region between the conjunctiva and the corneal epithelium, which have distinct characteristics77. In particular, the corneal epithelium has an extremely efficient regenerative capacity both in the normal homeostatic turnover of surface cells and in the response to injury.
Mesenchymal stem cells
The use of mesenchymal stem cells (MSCs) has been proposed in various autoimmune diseases, including uveitis. In a preclinical experimental model of uveitis performed in the rat, mesenchymal stem cells were shown to have a therapeutic effect78. The use of MSC has also been explored in ocular surface pathologies. In these studies79,80 the cells are administered intraperitoneally or intravenously. In addition, since they do not actually take root in the cornea, the cells are believed to act through the secretion of a protein with anti-inflammatory activity called TSG-6. Those just described are just a few examples in the literature where the use of mesenchymal stem cells is extended to ocular therapy. It is also evident that the inflammatory component still remains in the foreground as the main therapeutic target in this type of cell treatment.
Equally interesting is the potential use of cell therapy, targeting those inflammatory cells that damage the host tissue.
It has recently been shown that during the resolution phase of inflammation, accessory cells, such as the suppressor cells of derivation myeloid (MDSC) and regulatory T cells (Treg) are important not only as resolution factors, but also for their ability to link the innate immune system with the adaptive one81.
Myeloid-derived suppressor cells
Pro-inflammatory macrophages are important mediators of damage caused in intraocular inflammation82-84 and are the main cells to be blocked by anti-TNFa therapy85-87. However, as in all inflammatory processes, subsets of macrophages are activated during disease evolution, as was observed in an experimental autoimmune uveoretinitis (EAU) model, induced in C57/BL6 mice. In this model, the key role of myeloid-derived suppressor cells in the resolution of the inflammatory pathology is also demonstrated. Under these experimental conditions, there is also a high possibility of significant chronicity and subsequent retinal and subretinal neovascularisation. In view of the anti-inflammatory activity shown by MDSCs in different types of autoimmune disease88,89the use of MDSCs has also been proposed in chronic intraocular inflammation, given that it is under these circumstances that MDSCs expand and acquire anti-inflammatory activity through the release of suppressor cytokines90. Thus, MDSCs induced by retinal pigmented epithelium cells were shown to effectively block T-cell proliferation and the production of inflammatory cytokines. In addition, systemic administration of these cells inhibits the autoreactive T-cell responses responsible for retinal injury in the EAU model.91. In this model, retina-specific T cells cause local inflammation resulting in a breakdown of the blood-retinal barrier, infiltration of leucocytes and finally retinal detachment.92.
One of the main difficulties in the application of MDSCs in the control of intraocular inflammatory diseases is the insufficient availability of autologous cells. This is why research has definitely focused on finding a viable solution to overcome this critical point. It was not long ago that a study was published in which it was demonstrated that cultures of monocyte precursors, isolated from peripheral blood in the presence of prostaglandin E2, produce, in a simple and clinically acceptable manner, a high number of MDSC93. It has also been observed that MDSCs can be obtained in large quantities using cytokines from monocyte progenitor cells. These recent successes in developing new methods to generate MDSCs offer good opportunities to clinically test the efficacy of myeloid-derived suppressor cells in particular intraocular inflammatory diseases in the near future.
- Regulatory T cells
Regulatory T cells (Treg), a subpopulation of CD4+ CD25+ T lymphocytes co-expressing the transcription factor Foxp394regulate immune responses through the secretion of immunosuppressive and pro-response cytokines (IL10 and TGF-?), thus making them indispensable in restoring immune homeostasis95,96. Precisely because of this characteristic, the prospect of using these cells in the therapy of certain inflammatory diseases is a development that is considered quite logical and interesting. To date, the advantageous role of using Tregs has already emerged in a number of treatments of inflammatory diseases such as rheumatoid arthritis97multiple sclerosis98 and atherosclerosis99.
Three phase I clinical trials have recently been initiated to evaluate the absence of toxicity of Treg as a cell therapy in graft versus host disease (GvHD) with satisfactory results100,101,102. Treg administered in children with type I diabetes was able to slow the progression of the disease without causing serious adverse events103.
In the absence of clear Treg toxicity and in view of the fact that patients with active uveitis have a reduced level of circulating Treg104-106The clinical application of Treg cells has also been proposed in the treatment of uveitis. This is also supported by a series of experimental in vivo treatments showing that good efficacy in controlling intraocular inflammation can be achieved through the use of Treg cells107,108. A difficulty encountered in translating Treg therapy into clinical practice was mainly related to the high frequency of activated conventional T cells present in the CD4+CD25+ fraction and the limited availability of standard Good Manufacturing Practice (GMP) procedures for removing cellular contaminants109. Another limitation for their clinical application was associated with the small number of Treg available in the transfer phase.110,102. Various approaches have been taken for selective expansion of human Treg cells111-114. However, only recently115 a robust procedure for the production of Treg for clinical use has been reported. The latter results look very promising and could initiate cell therapy with Treg for the effective modulation of chronic intraocular inflammation in the near future.
Conclusions
In recent decades, various therapeutic approaches have been developed for the treatment of eye diseases previously considered difficult to cure. Part of this success is mainly due to the many experimental evidences that have identified the inflammatory process as playing a determining and/or triggering role in various eye diseases. Gradually, the field of pharmacological/clinical intervention has opened up to new therapeutic solutions, many of which have already been consolidated in clinical practice, while others are still in the process of being examined or are at an early stage of ophthalmic experimentation.
A path has been mapped out and it is to be hoped, based on the scenario analysed so far, that in the future the opportunities for successfully controlling the ocular inflammatory process will be greater than in the past.
Antonino Asero
E-mail: Antonino.Asero@sifigroup.com
Bibliography
1. Streilein JW. Ocular immune privilege: therapeutic opportunities from an experiment of nature. Nat. Rev. Immunol. 2003 Nov;3(11):879-89.
2. McDermott, Perez VHuang AJ, et al. 2005. Pathways of corneal and ocular surface inflammation: a perspective from the Cullen symposium. Ocul. 2005 Oct;3(4 Suppl):S131-8
3. Niederkorn JY, Stein-Streilein J. History and physiology of immune privilege. Ocul. Immunol. Inflamm. 2010 Jan;18(1):19-23.
4. Cheung CM, Vania M, Ang M, et al. Comparison of aqueous humor cytokine and chemokine levels in diabetic patients with and without retinopathy. Molecular Vision. 2012; 18:830-837.
5. Gronert K. Resolution, the grail for healthy ocular inflammation. Exp. Eye Res. 2010 Oct;91(4):478-85.
6. Alessandri AL, Sousa LP, Lucas CD, et al. Resolution of inflammation: mechanisms and opportunities for drug development. Pharmacol Ther. 2013 Aug;139(2):189-212
7. Gilroy DW, Lawrence T, Perretti M, et al. Inflammatory resolution: new opportunities for drug discovery. Nat Rev Drug Discov. 2004;3(5):401-16.
8. Rossi AG, Hallett JM, Sawatzky DA, et al. Modulation of granulocyte apoptosis can influence the resolution of inflammation. Biochem Soc Trans. 2007 Apr;35(Pt 2):288-91.
9. Hallett JM, Leitch AE, Riley NA, et al. Novel pharmacological strategies for driving inflammatory cell apoptosis and enhancing the resolution of inflammation. Trends Pharmacol Sci. 2008;29(5):250-7.
10. Serhan CN, Brain SD, Buckley CD, et al. Resolution of inflammation: state of the art, definitions and terms. FASEB J. 2007. 21, 325-332.
11. Duffin R, Leitch AE, Fox S. Targeting granulocyte apoptosis: mechanisms, models, and therapies. Immunol Rev. 2010 Jul;236:28-40.
12. Serhan CN. Resolution phase of inflammation: novel endogenous anti-inflammatory and proresolving lipid mediators and pathways. Annu Rev Immunol. 2007;25:101-37.
13. Serhan CN, Chiang N. Endogenous pro-resolving and anti-inflammatory lipid mediators: a new pharmacologic genus. Br J Pharmacol. 2008 Mar;153 Suppl 1:S200-S215.
14. Serhan CN, Chiang N, Van Dyke TE. Resolving inflammation: dual anti-inflammatory and pro-resolution lipid mediators. Nat Rev Immunol. 2008 May;8(5):349-61.
15. Serhan CN, Petasis NA, 2011. Resolvins and protectins in inflammation resolution. Chem Rev. 2011 Oct 12;111(10):5922-43.
16. Levy BD, Zhang QYBonnans C, et al. The endogenous pro-resolving mediators lipoxin A4 and resolvin E1 preserve organ function in allograft rejection. Prostaglandins Leukot Essent Fatty Acids. 2011;84(1-2):43-50.
17. Souza DG, Fagundes CT, Amaral FA, et al. The required role of endogenously produced lipoxin A4 and annexin-1 for the production of IL-10 and inflammatory hyporesponsiveness in mice. J Immunol. 2007;15;179(12):8533-43.
18. Conte FP, Menezes-de-Lima O Jr, Verri WA Jr, et al. Lipoxin A(4) attenuates zymosan-induced arthritis by modulating endothelin-1 and its effects. Br J Pharmacol. 2010;161(4):911-24.
19. Serhan CN, Yang R, Martinod K, et al. Maresins: novel macrophage mediators with potent antiinflammatory and proresolving actions. J Exp Med. 2009;206, 15-23.
20. Schwab JM, Chiang N, Arita M, et al. Resolvin E1 and protectin D1 activate inflammation-resolution programmes. Nature Letters, 2008;447:869-75.
21. Chiang N, Fredman G, Backhed F, et al. Infection regulates pro-resolving mediators that lower antibiotic requirements. Nature. 2012;484: 524-528.
22. Xu ZZ, Zhang L, Liu T, et al. Resolvins RvE1 and RvD1 attenuate inflammatory pain via central and peripheral actions. Nat Med. 2010;16(5):592-7, 1p following 597.
23. Serhan CN, Hamberg M, Samuelsson B. Lipoxins: novel series of biologically active compounds formed from arachidonic acid in human leukocytes. Proc Natl Acad Sci USA. 1984; 81(17): 5335-5339.
24. Chiang N, Arita M, Serhan CN. Anti-inflammatory circuitry: lipoxin, aspirin-triggered lipoxins and their receptor ALX. Prostaglandins Leukot Essent Fatty Acids. 2005;73(3-4):163-77.
25. Chiang N, Serhan CN, Dahlén SE, et al. The lipoxin receptor ALX: potent ligand-specific and stereoselective actions in vivo. Pharmacol Rev. 2006;58(3):463-87.
26. Godson C, Brady HR. Lipoxins: novel anti-inflammatory therapeutics? Curr Opin Investig Drugs. 2000;1(3):380-5.
27. Maderna P, Godson C, Taking insult from injury: lipoxins and lipoxin receptor agonists and phagocytosis of apoptotic cells. Prostaglandins Leukot Essent Fatty Acids. 2005 Sep-Oct;73(3-4):179-87.
28. Fierro IM, Colgan SP, Bernasconi G, et al. Lipoxin A4 and aspirin-triggered 15-epi-lipoxin A4 inhibit human neutrophil migration: comparisons between synthetic 15 epimers in chemotaxis and transmigration with microvessel endothelial cells and epithelial cells. J Immunol. 2003 Mar 1;170(5):2688-94.
29. Gronert K. Lipoxins in the eye and their role in wound healing. Prostaglandins Leukot Essent Fatty Acids. 2005 Sep-Oct;73(3-4):221-9.
30. Bazan NG. Cell survival matters: docosahexaenoic acid signaling, neuroprotection and photoreceptors. Trends Neurosci. 2006;29,263- 271.
31. Biteman B, Hassan IR, Walker E, et al. Interdependence of lipoxin A4 and heme-oxygenase in counter-regulating inflammation during corneal wound healing. FASEB J. 2007 Jul;21(9):2257-66.
32. Qin Q, Patil KA, Gronert K, et al. Neuroprotectin D1 inhibits retinal ganglion cell death following axotomy. Prostaglandins Leukot. Essent. Fatty Acids. 2008; 79, 201-207.
33. Bazan NG, 2009. Neuroprotectin D1-mediated anti-inflammatory and survival signaling in stroke, retinal degenerations, and Alzheimer's disease. J. Lipid. Res. 2009 Apr;50 (Suppl.), S400-S405
34. Calandria JM, Marcheselli VL, Mukherjee PK, et alSelective survival rescue in 15-lipoxygenase-1 deficient retinal pigment epithelial cells by the novel docosahexaenoic acid-derived mediator, neuroprotectin D1. J. Biol. Chem. 2009; 284, 17877-17882.
35. Kenchegowda S, Bazan HE. Significance of lipid mediators in corneal injury and repair. J Lipid Res. 2010 May;51(5):879-91.
36. Medeiros R, Rodrigues GB, Figueiredo CP, et al. Molecular mechanisms of topical anti-inflammatory effects of lipoxin A(4) in endotoxin-induced uveitis. Mol Pharmacol. 2008;74(1):154-61.
37. József L, Zouki C, Petasis NA, et al. Lipoxin A4 and aspirin-triggered 15-epi-lipoxin A4 inhibit peroxynitrite formation, NF-kappa B and AP-1 activation, and IL-8 gene expression in human leukocytes. Proc Natl Acad Sci U S A. 2002 Oct 1;99(20):13266-71.
38. Rodgers K, McMahon B, Mitchell D, et al. Lipoxin A4 modifies platelet-derived growth factor-induced pro-fibrotic gene expression in human renal mesangial cells, Am. J. Pathol. 2005;167 683-694.
39. Martins V, Valença SS, Farias-Filho F., et al. ATLa, an aspirin-triggered lipoxin A4 synthetic analog, prevents the inflammatory and fibrotic effects of bleomycin-induced pulmonary fibrosis, J. Immunol. 2009;182 5374-5381.
40. Guilford WJ, Parkinson JF Second-generation beta-oxidation-resistant 3-oxa-lipoxin A4 analogs. Prostaglandins Leukot Essent Fatty Acids. 2005 Sep-Oct;73(3-4):245-50.
41. Serhan CN. The resolution of inflammation: the devil in the flask and in the details. The FASEB Journal. 2011 May; 25(5)1441-1448.
42. Haworth O, Cernadas M, Yang R, et al. Resolvin E1 regulates interleukin-23, interferon-gamma and lipoxin A4 to promote resolution of allergic airway inflammation. Nat Immunol 2008, 9:873-879.
43. Li N, He J, Schwartz CE, et al. Resolvin E1 improves tear production and decreases inflammation in a dry eye mouse model. J. Ocul. Pharmacol. Ther, 26, 431-439 (2010).
44. Zhang F, Yang H, Pan Z, et al. Dependence of resolvin-induced increases in corneal epithelial cell migration on EGF receptor transactivation. Invest Ophthalmol Vis Sci (2010b). 51, 5601-5609.
45. Dartt DA, Hodges RR, Li D, et al. Conjunctival Goblet Cell Secretion Stimulated by Leukotrienes Is Reduced by Resolvins D1 and E1 To Promote Resolution of Inflammation. J Immunol. 2011 Apr 1;186(7):4455-66.
46. de Paiva CS, Schwartz CE, Gjorstrup P, et al. Resolvin E1 (RX-10001) reduces corneal epithelial barrier disruption and protects against goblet cell loss in a murine model of dry eye. Cornea 2012;31:1299-1303.
47. Li D, Hodges RR, Jiao J, et al. Resolvin D1 and aspirin-triggered resolvin D1 regulate histamine-stimulated conjunctival goblet cell secretion. Mucosal Immunol. 2013;6(6):1119-30.
48. Krishnamoorthy S, Recchiuti A, Chiang N, et al. Resolvin D1 Receptor Stereoselectivity and Regulation of Inflammation and Proresolving MicroRNAs. Am J Pathol. 2012; 180(5): 2018-2027.
49. Tian H, Lu Y, Sherwood AM, et al. Resolvins E1 and D1 in Choroid-Retinal Endothelial Cells and Leukocytes: Biosynthesis and Mechanisms of Anti-inflammatory Actions. Invest. Ophthalmol. Vis. Sci. 2009; 50(8):3613-20.
50. Marcheselli VL, Hong S, Lukiw WJ, et al. Novel docosanoids inhibit brain ischemia-reperfusion-mediated leukocyte infiltration and pro-inflammatory gene expression. J Biol Chem. 2003. 278, 43807-43817.
51. Bannenberg GL, Chiang N, Ariel A, et al. Molecular circuits of resolution: formation and actions of resolvins and protectins. J Immunol. 2005; 174, 4345-4355.
52. Duffield JS, Hong S, Vaidya VS, et al. Resolvin D series and protectin D1 mitigate acute kidney injury. J Immunol. 2006;177, 5902-5911.
53. Levy BD, Kohli P, Gotlinger K, et al. Protectin D1 is generated in asthma and dampens airway inflammation and hyperresponsiveness. J Immunol. 2007. 178, 496-502.
54. Bazan NG. Neuroprotectin D1 (NPD1): a DHA-derived mediator that protects brain and retina against cell injury-induced oxidative stress. Brain Pathol. 2005;15(2):159-66.
55. Mukherjee PK, Marcheselli VL, Serhan CN, et al. Neuroprotectin D1: A docosahexaenoic acid-derived docosatriene protects human retinal pigment epithelial cells from oxidative stress. PNAS, 2004;101(22):8491-8496.
56. Calandria JM, Mukherjee PK, de Rivero Vaccari JC, et al. Ataxin-1 Poly(Q)-induced proteotoxic stress and apoptosis are attenuated in neural cells by docosahexaenoic acid-derived Neuroprotectin D1. J Biol Chem, 2012;287:23726-39.
57. Cortina MS, He J, Russ T, et al. Neuroprotectin D1 restores corneal nerve integrity and function after damage from experimental surgery. Invest Ophthalmol Vis Sci. 2013;54:4109-4116.
58. Serhan CN, Dalli J, Karamnov S, et al. Macrophage proresolving mediator maresin 1 stimulates tissue regeneration and controls pain. PHASEB J. 2012;26(4):1755-65.
59. Lacotte S, Brun S, Muller S, et al. CXCR3, inflammation, and autoimmune diseases. Ann N Y Acad Sci. 2009;1173:310-7.
60. Wijtmans M, Verzijl D, Leurs R, et al. Towards small-molecule CXCR3 ligands with clinical potential. Chem Med Chem 2008, 3:861-872.
61. Crosignani S, Missotten M, Cleva C, et al. Discovery of a novel series of CXCR3 antagonists. Bioorg Med Chem Lett 2010, 20:3614-3617.
62. Jenh CH, Cox MA, Cui L, et al.: A selective and potent CXCR3 antagonist SCH 546738 attenuates the development of autoimmune diseases and delays graft rejection. BMC Immunol. 2012 Jan 10;13(1):2.
63. Yoon KC, Park CS, You IC, et al. Expression of CXCL9, -10, -11, and CXCR3 in the tear film and ocular surface of patients with dry eye syndrome. Invest Ophthalmol Vis Sci. 2010;51: 643-650.
64. Coursey TG., Gandhi NB, Volpe EA, et al. Chemokine Receptors CCR6 and CXCR3 Are Necessary for CD4+ T Cell Mediated Ocular Surface Disease in Experimental Dry Eye Disease. Plos One. 2013;8;11, e78508.
65. Perez VL, Saeed AM, Tan Y, et al. The eye: A window to the soul of the immune system. J Autoimmun. 2013 Sep;45:7-14.
66. Gao P, Zhou XY, Yashiro-Ohtani Y, et al. The unique target specificity of a nonpeptide chemokine receptor antagonist: selective blockade of two Th1 chemokine receptors CCR5 and CXCR3. J Leukoc Biol 2003;73:273-80.
67. Sennlaub F, Auvynet C, Calippe B, et al. CCR2(+) monocytes infiltrate atrophic lesions in age-related macular disease and mediate photoreceptor degeneration in experimental subretinal inflammation in Cx3cr1 deficient mice. EMBO Mol Med. 2013 Nov;5(11):1775-93
68. Sousa LP, Alessandri AL, Pinho V, et al. Pharmacological strategies to resolve acute inflammation. Current Opinion in Pharmacology, 2013; 13: 1-7.
69. Teixeira MM, Gristwood RW, Cooper N, et al. Phosphodiesterase (PDE)4 inhibitors: anti-inflammatory drugs of the future? Trends Pharmacol Sci 1997, 18:164-171.
70. Sousa LP, Carmo AF, Rezende BM, et al. Cyclic amp enhances resolution of allergic pleurisy by promoting inflammatory cell apoptosis via inhibition of PI3K/ Akt and NF-kappaB. Biochem Pharmacol 2009, 78:396-405.
71. Sousa LP, Lopes F, Silva DM, et al. PdE4 inhibition drives resolution of neutrophilic inflammation by inducing apoptosis in a PKA-PI3K/Akt- dependent and NF-kappa B-independent manner. J Leukoc Biol 2010, 87:895-904.
72. Bystrom J, Evans I, Newson J, et al. Resolution-phase macrophages possess a unique inflammatory phenotype that is controlled by cAMP. Blood 2008, 112:4117-4127.
73. Rajakariar R, Newson J, Jackson EK, et al. Nonresolving inflammation in gp91phoxS/S mice, a model of human chronic granulomatous disease, has lower adenosine and cyclic adenosine 50-monophosphate. J Immunol 2009, 182:3262-3269.
74. Gale DC, Sychterz CJ, Rodgers C, et al. Ocular and Systemic Pharmacokinetics of a PDE4 Inhibitor Following Topical Administration (Eyedrop) in Male Dutch-Belted Rabbits. ARVO abstract no. 5060, 2013.
75. Forrester JV, Steptoe RJ, Klaska IP, et al. Cell-based therapies for ocular inflammation. Prog Retin Eye Res. 2013 Jul;35:82-101.
76. Medina RJ, Archer DB, Stitt, AW. Eyes open to stem cells: safety trial may pave the way for cell therapy to treat retinal disease in patients. Stem Cell Res Ther. 2011 Dec 7;2(6):47.
77. Vasania VS, Prasad P, Gill RK, et al. Molecular and cellular characterization of expanded and cryopreserved human limbal epithelial stem cells reveal unique immunological properties. Exp. Eye Res. 2011;92, 47-56.
78. Zhang, X, Ren X, Li G, et al. Mesenchymal stem cells ameliorate experimental autoimmune uveoretinitis by comprehensive modulation of systemic autoimmunity. Invest. Ophthalmol. Vis. Sci. 2011; 52, 3143-3152.
79. Oh JY, Roddy GW, Choi H, et al. Anti-inflammatory protein TSG-6 reduces inflammatory damage to the cornea following chemical and mechanical injury. Proc. Natl. Acad. Sci. U. S. A. 2010; 107, 16875-16880.
80. Roddy GW, Oh JY, Lee R, et al. Action at a distance: systemically administered adult stem/progenitor cells (MSCs) reduce inflammatory damage to the cornea without engraftment and primarily by secretion of TNF-alpha stimulated gene/protein 6. Stem Cells. 2011;29, 1572-1579.
81. Ortega-Gómez A, Perretti M, Soehnlein O. Resolution of inflammation: an integrated view EMBO Mol Med. 2013; 5(5): 661-674.
82. Forrester JV, Mcmenamin PG, Holthouse I, et al. Localization and characterization of major histocompatibility complex class IIpositive cells in the posterior segment of the eye: implications for induction of autoimmune uveoretinitis. Invest. Ophthalmol. Vis. Sci. 1994; 35, 64-77.
83. Forrester JV, Huitinga I, Lumsden L, et al. Marrow-derived activated macrophages are required during the effector phase of experimental autoimmune uveoretinitis in rats. Curr. Eye Res. 1998;17, 426-437.
84. Jiang HR, Lumsden L, Forrester JV. Macrophages and dendritic cells in IRBP-induced experimental autoimmune uveoretinitis in B10RIII mice. Invest. Ophthalmol. Vis. Sci. 1999;40, 3177-3185.
85. Dick AD, Mcmenamin PG, Korner H, et al. Inhibition of tumor necrosis factor activity minimizes target organ damage in experimental autoimmune uveoretinitis despite quantitatively normal activated T cell traffic to the retina. Eur. J. Immunol. 1996; 26,1018-1025.
86. Dick AD, Carter D, Robertson M, et al. Control of myeloid activity during retinal inflammation. J Leukoc Biol. 2003 Aug;74(2):161-6.
87. Dick AD, Forrester JV, Liversidge J, et al. The role of tumour necrosis factor (TNF-alpha) in experimental autoimmune uveoretinitis (EAU). Prog. Retin. Eye Res. 2004;23, 617-637.
88. Zhu B, Bando Y, Xiao S, et al. CD11b+ Ly-6C(hi) suppressive monocytes in experimental autoimmune encephalomyelitis. J. Immunol. 2007; 179, 5228-5237.
89. Moline-Velazquez V, Cuervo H, Vila-Del Sol V, et al. Myeloid-derived suppressor cells limit the inflammation by promoting T lymphocyte apoptosis in the spinal cord of a murine model of multiple sclerosis. Brain Pathol. 2011; 21, 678-691.
90. Ray P, Arora M, Poe SL, et al. Lung myeloid-derived suppressor cells and regulation of inflammation. Immunol. Res. 2011; 50, 153-158.
91. Tu Z, Li Y, Smith D, et al. Myeloid suppressor cells induced by retinal pigment epithelial cells inhibit autoreactive T cell responses that lead to experimental autoimmune uveitis. Invest. Ophthalmol. Vis. Sci. 2012; 53, 959-966.
92. Kerr EC, Raveney BJ, Copland DA, et al. Analysis of retinal cellular infiltrate in experimental autoimmune uveoretinitis reveals multiple regulatory cell populations. J Autoimmun. 2008;31:354-361.
93. Obermajer N, Kalinski P. Generation of myeloid-derived suppressor cells using prostaglandin E2. Transplantation Research 2012, 1:15.
94. Hori S, Nomura T, Sakaguchi S. Control of regulatory T cell development by the transcription factor Foxp3. Science. 2003;299:1057-1061.
95. Asseman C, Mauze S, Leach MW, et al. An essential role for interleukin 10 in the function of regulatory T cells that inhibit intestinal inflammation. J Exp Med .1999;190:995-1004.
96. Fahlen L, Read S, Gorelik L, et al. T cells that cannot respond to TGF-beta escape control by CD4(+)CD25(+) regulatory T cells. J Exp Med. 2005; 201: 737-746.
97. Cao D, Malmstrom V, Baecher-Allan C, et al. Isolation and functional characterization of regulatory CD25brightCD4+ T cells from the target organ of patients with rheumatoid arthritis. Eur J Immunol. 2003; 33: 215-223.
98. Viglietta V, Baecher-Allan C, Weiner HL, et al. Loss of functional suppression by CD4+CD25+ regulatory T cells in patients with multiple sclerosis. J Exp Med. 2004;199: 971-979.
99. Ait-Oufella H, Salomon BL, Potteaux S, et al. Natural regulatory T cells control the development of atherosclerosis in mice. Nat Med. 2006;12: 178-180.
100. Trzonkowski P, Bieniaszewska M, Juscinska J, et al. First-in-man clinical results of the treatment of patients with graft versus host disease with human ex vivo expanded CD4+CD25+CD127-T regulatory cells. Clin Immunol 2009;133(1):22-26.
101. Brunstein CG, Miller JS, Cao Q, et al. Infusion of ex vivo expanded T regulatory cells in adults transplanted with umbilical cord blood: Safety profile and detection kinetics. Blood 2010; 117:1061-1070.
102. Di Ianni M, Falzetti F, Carotti A, et al. Tregs prevent GVHD and promote immune reconstitution in HLA-haploidentical transplantation. Blood. 2011; 117: 3921-3928.
103. Marek-Trzonkowska N, My?liwec M, Siebert J, et al. Clinical application of regulatory T cells in type 1 diabetes. Pediatr Diabetes. 2013;14(5):322-32.
104. Chen L, Yang P, Zhou H, et al. Diminished frequency and function of CD4+CD25 high regulatory T cells associated with active uveitis in Vogt-Koyanagi-Harada syndrome. Invest. Ophthalmol. Vis. Sci. 2008; 49, 3475-3482.
105. Nanke Y, Kotake S, Goto M, et al. Decreased percentages of regulatory T cells in peripheral blood of patients with Behcet's disease before ocular attack: a possible predictive marker of ocular attack. Mod. Rheumatol. 2008; 18, 354-358.
106. Yeh S, Li Z, Forooghian F, et al. CD4+Foxp3+ T-regulatory cells in noninfectious uveitis. Arch Ophthalmol. 2009 Apr;127(4):407-13.
107. Larson T, Nussenblatt RB, Sen HN. Emerging drugs for uveitis. Expert Opin. Emerg. Drugs. 2011;16, 309-322.
108. Calleja S, Cordero-Coma M, Rodriguez E, et al. Adalimumab specifically induces CD3(+) CD4(+) CD25(high) Foxp3(+) CD127(-) T-regulatory cells and decreases vascular endothelial growth factor plasma levels in refractory immune-mediated uveitis: a non-randomized pilot intervention study. Eye (Lond) 2012: 26, 468-477.
109. Riley JL, June CH, Blazar BR. Human T Regulatory Cells as Therapeutic Agents: Take a Billion or So of These and Call Me in the Morning. Immunity. 2009; 30(5): 656-665.
110. Brunstein CG, Fuchs EJ, Carter SL, et al. Alternative donor transplantation after reduced intensity conditioning: results of parallel phase 2 trials using partially HLA-mismatched related bone marrow or unrelated double umbilical cord blood grafts. Blood. 2011;118, 282-288.
111. Sagoo P, Ali N, Garg G, et al. Human regulatory T cells with alloantigen specificity are more potent inhibitors of alloimmune skin graft damage than polyclonal regulatory T cells. Sci Transl Med 2011; 3: 83ra42.
112. Peters JH, Hilbrands LB, Koenen HJ, et al. Ex vivo generation of human alloantigen-specific regulatory T cells from CD4(pos) CD25(high) T cells for immunotherapy. PLoS ONE 2008; 3: 2233.
113. Koenen HJ, Fasse E, Joosten I. CD27/CFSE-based ex vivo selection of highly suppressive alloantigen-specific human regulatory T cells. J Immunol 2005; 174: 7573-7583.
114. Banerjee DK, Dhodapkar MV, Matayeva E, et al. Expansion of FOXP3high regulatory T cells by human dendritic cells (DCs) in vitro and after injection of cytokinematured DCs in myeloma patients. Blood 2006; 108: 2655-2661.
115. Putnam AL, Safinia N, Medvec A, et al. Tang. Clinical Grade Manufacturing of Human Alloantigen-Reactive Regulatory T Cells for Use in Transplantation. American Journal of Transplantation 2013;13(11):3010-20.
Dr. Carmelo Chines
Direttore responsabile